Journal of Transplantation & Stem Cell Biology
Download PDF
Review Article
*Address for Correspondence: Yong Li, Department of Pediatric Surgery, University of Texas Medical School at Houston, TX77030, USA; E-mail: yong.li.1@uth.tmc.edu
Citation: Vojnits K, Li Y. The current knowledge of immune privilege in stem cells. J Transplant Stem Cel Biol. 2014;1(1): 4.
Copyright © 2014 Vojnits K, et al. This is an open access article distributed under the Creative Commons Attribution License, which permits unrestricted use, distribution, and reproduction in any medium, provided the original work is properly cited.
Journal of Transplantation & Stem Cell Biology |ISSN 2374-9326 | Volume: 1, Issue: 1
Submission: 29 January 2014 | Accepted: 27 March 2014 | Published: 31 March 2014
Figure 1: Schematic diagram how immune privilege can be theoretically achieved by transplanted SCs. Immune privileged adult SCs express TGF-β and IL-10 that can activate regulatory T cells (Treg-cells). Treg-cells can actively prevent T cells from activation, and also may polarize them to a regulatory phenotype. Fas-ligand (FasL) expression on SCs can also prevent immune responses by inducing apoptosis in Fas-bearing leukocytes. Natural killer cells (NK cell) are cytotoxic lymphocytes that affect adaptive immune responses. NK cell facilitated cell lysis is regulated by the balance of stimulatory and inhibitory signals that they recognize on target cells. NK cell inhibitory ligands include members of the major histocompatibility complex (MHC) family, such as MHC I. If MHC I is present on target cells, it inhibits NK cells’ activation. Although, most adult SCs express only low level of MHC I, it still can sufficiently inhibit the function of NK cells. Furthermore, adult SCs are also protected from immune rejection when they lack the expression of stimulatory signals for NK cells.
The current knowledge of immune privilege in stem cells
Kinga Vojnits1,2 and Yong Li1,2*
- 1Department of Pediatric Surgery, University of Texas Medical School at Houston, TX77030, USA
- 2Center for Stem Cell and Regenerative Medicine, University of Texas Health Science Center at Houston (UT Health), TX77030, USA
*Address for Correspondence: Yong Li, Department of Pediatric Surgery, University of Texas Medical School at Houston, TX77030, USA; E-mail: yong.li.1@uth.tmc.edu
Citation: Vojnits K, Li Y. The current knowledge of immune privilege in stem cells. J Transplant Stem Cel Biol. 2014;1(1): 4.
Copyright © 2014 Vojnits K, et al. This is an open access article distributed under the Creative Commons Attribution License, which permits unrestricted use, distribution, and reproduction in any medium, provided the original work is properly cited.
Journal of Transplantation & Stem Cell Biology |ISSN 2374-9326 | Volume: 1, Issue: 1
Submission: 29 January 2014 | Accepted: 27 March 2014 | Published: 31 March 2014
Abstract
Immune privilege as an active defensive mechanism guards cells and tissues against foreign antigens. Developing tumors and adult stem cells are believed to be immune privileged, since they have the ability to avoid rejection after implantation. Allograft rejection of transplanted tissues and organs occurs because of allelic differences between graft and host at polymorphic loci, which give rise to histocompatibility antigens; unless immunosuppressive therapy is given. This obstacle also exists when using human embryonic stem cell for cell transplantation, rising challenges in cell therapy and in regenerative medicine. Therefore, to better understand the mechanisms underlying in immune privilege and to obtain strategies which can mimic the naturally immune privileged cells, is of utmost significance in transplantation and regenerative medicine.Keywords
Adult stem cell; Immune privilege; Interleukin-10; Transforming growth factor-β (TGF-β); Major histocompatibility Complex(MHC)Regulatory Molecules in Immune Privilege
Immune privilege, providing protection to the cells and tissues of the body against foreign antigens, can be acquired locally in many different tissues in response to inflammation [1,2]. It is regulated at multiple levels including small molecules, (e.g., amino acids) intracellular enzymes, e.g. indoleamine 2,3-dioxygenase, and major histocompatibility complex (MHC) molecules [3,4]. MHC antigen expression is an important mediator of the adaptive immune response, and is essential for the recognition of both self- and foreign antigens [5]. The down regulation of MHC I and II antigen production is a common feature shared by many immune privileged tissues and cells. However, this is not enough to achieve complete immune privilege, as cells that lack MHC I expression are still vulnerable to the natural killer (NK) cell response. Therefore, many of the immune privileged tissues actively suppress NK cell responses [6]. Other players in immune privilege are surface expressed regulatory factors, like Fasligand (FasL) and programmed death-1 receptor (B7-H1) [7], as well as membrane complement regulatory proteins [8].Role of TGF-β in Immune Privilege
Immune privilege is a complex process (Figure 1). The immune privileged cells often express immunoregulatory and antiinflammatory factors, such as, interleukin-10 and transforming growth factor-β (TGF-β), which act locally to create an immune privileged site for the cells [9,10]. TGF-β, a highly conserved regulatory cytokine, is expressed by most of the cells [11]. It has pleiotropic effects on cell proliferation, differentiation, migration and survival and plays a key role in development, differentiation, tumorigenesis, fibrosis, wound healing and also as a switch factor in locoregional immune suppression [12,13]. TGF-β is also able to inhibit MHC antigen expression in HeLa cells [14]. In the immune system, TGF-β functions as a referee of chemotaxis, activation, and survival of lymphocytes, NK cells, dendritic cells, macrophages, mast cells, and granulocytes, and by that it maintains immune tolerance. From the members of the TGF-β receptor family, TGF-β1 represents the dominant mediator of immune and inflammatory events. It contributes to the function and generation of regulatory T cells [15] which can further facilitate inflammatory and immune reactions. TGF-β is also expressed in multiple immune privileged sites contributing to immune privilege in each of these sites, e.g. in anterior chamber of the eye, central nervous system, adrenal cortex, testes, and in the vitreous humor [16]. Moreover, it has been previously reported that TGF-β directly promotes immune privilege to mesenchymal SCs by contributing to the inhibition of T cell proliferation [17,18] via suppression of c-Myc and enhancement of cell cycle inhibitors, such as p15INK4b and p21CIP1 [19]. The further role or contribution of TGF-β regarding to immune protection to other SCs has not been revealed yet. Interleukin-10, the other cytokine which can be secreted by regulatory T cells, is produced by many cell types, such as keratinocytes, B cells, mast cells and monocytes [20-23]. Since its discovery, it has been proven that interleukin-10 has a profound effect on cell-mediated immune responses, and it also limits the induction of inflammation [16]. However, the mechanism of immune privilege acquired by regulatory T cells-derived interleukin-10 remains unclear. Furthermore, TGF-β itself also induces interleukin-10 secretion, and interleukin-10 is able to motivate TGF-β; therefore it directly or indirectly interacts with the immune privilege processes.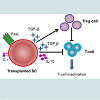
Stem Cells and Immune Privilege
Adult stem cells (SCs), as undifferentiated cells residing in several tissues and organs, are required for the growth, routine turnover, and rejuvenation of tissues as well as for their response to injury. They can self-renew and have multiple differentiation capacities into the major specialized cell types of the tissue in which they reside. Adult SCs are typically long-lived and quiescent cells which can be activated for proliferation and differentiation by stress or tissue injury. Various adult stem cell populations, such as mesenchymal SCs [24-27], hematopoietic SCs [28], neural SCs [29], amnion SCs [30], andmuscle SCs [31], are immune privileged to a certain extent. They have to be protected from adverse autoimmune responses or local damage from chronic inflammation and it is thought that besides their own immune privilege, their native niche can serve as a protective microenvironment [5].Mesenchymal SCs are derived and isolated from various tissues including adipose tissue and bone marrow, and they can differentiate into adipogenic, osteogenic and chondrogenic lineages, among others [32]. They are well known for their capacity to suppress inflammation and inhibit the immune response [24], which came from the first observation that bone marrow-derived mesenchymal SCs suppressed T cell proliferation [33,34]. Mesenchymal SCs express no MHC II, low levels of MHC Ia, and high levels of MHCIb. Mesenchymal SCs niche, formed by mesenchymal SCs and the surrounding stromal cells, inhibits the cyclin-D2 expression which disrupts the cell cycle of T cells [25,35]. Moreover, in response to increased interferon-γ production by T cells, mesenchymal SCs express B7-H1, which can down-modulate the effector functions of activated T cells [36]. The immune privilege of mesenchymal SCs has already been utilized in tissue repair and in prevention of graft-versus-host diseases [37]. For example, transplanted mesenchymal SCs could block degeneration of intervertebral discs in a surgically induced canine nucleotomy model by contributing to the maintenance of immune privilege via FasL production [38]. However, recent clinical trials and studies show that transplanted mesenchymal SCs can be allo-rejected, therefore they are not ultimately immune privileged as it has been shown before [39]. Rather, They can be called as “immune evasive” [39].
Hematopoietic SCs give rise to myeloid and lymphoid lineages and have been widely used in transplantation to treat patients with leukemia, lymphoma, some solid cancers, and autoimmune diseases [40]. It has already been shown that the niche where hematopoietic SCs reside provides an immune privileged site for them against foreign antigens [28]. This natural in vivo microenvironment for hematopoietic SCs is formed by stromal cells, osteoblasts, and sinusoidal endothelial cells, among others, and controls hematopoietic SCs quiescence, apoptosis, migration, as well as cell division. It has recently been shown that regulatory T cells colocalize with hematopoietic SCs in the endosteal area of the bone marrow, providing an immune privileged site for them [41]. Moreover, hematopoietic SCs are also capable of interacting with the immune system as signal “receivers” via toll-like receptors, tumor necrosis factor α receptor, interferon receptors [42]; and as signal “providers” mediated by surface immune inhibitory molecules, such as CD47 and CD247 [43], when they are out of their niche. This inhibits attack from the innate and adaptive immune responses, similarly to the process of immune privilege of mesenchymal and amnion SCs.
Muscle SCs - also considered also as satellite cells or a subpopulation of satellite cells - are a group of mononucleated cells located between the basal lamina and sarcolemma of muscle fibers [44-46]. Muscle SCs are the main players in skeletal muscle regeneration, and they also contribute to the postnatal growth of muscle fibers. Most muscle SCs express the paired domain transcription factor Pax7, myogenic regulatory factor Myf5, cell adhesion protein M-Cadherin, tyrosine receptor kinase c-Met, and chemokine receptor Cxcr4, among others [47,48]. Muscle SCs have been investigated for their notable multi-lineage differentiation capacity, self-renewal ability and immune privileged behavior [31]. Muscle SCs lack the expression of MHC I, and it was demonstrated that they displayed an improved transplantation capacity in skeletal muscle, and the injected cells were not rejected by the host allogeneic tissues [31].
Neural SCs are immune privileged not only in their native niche, but also in terms of allogeneic transplantation into a non-privileged site [5]. It was demonstrated that neural SCs and neural progenitor cells retained inherent immune privilege [29,49] when they were transplanted beneath the kidney capsule in the form of neurospheres. The transplanted allogeneic neural SCs survived at least 4 weeks, and they neither sensitized their hosts nor expressed detectable levels of major MHC class I or II.
In contrast to adult SCs, it appears that pluripotent embryonic SCs and induced pluripotent SCs are not immune privileged [50]. The immune properties of pluripotent SCs have been attracting research interest since it has been observed that embryonic SCs express low levels of MHC class I and hardly any MHC class II proteins [51]. Indeed, several reports have shown that both mouse and human embryonic SCs can avoid immune rejection, and mouse embryonic SCs can survive in immunocompetent mice, rats, and sheep for weeks [52-56]. However, it has has also been clearly demonstrated by recent studies that undifferentiated human embryonic SCs, embryonic SC-derived progenitors and induced pluripotent SCs can trigger an immune response even in syngeneic recipients and can be rejected upon transplantation [57-60]. These contradictory findings may be as consequences of the used evaluation methods for the survival of transplanted SCs, e.g. histological techniques vs. in vivo molecular imaging [61]. The latest studies provide evidences that the rejection of pluripotent SCs is mediated by CD4+ T cells [57], and that pluripotency genes, such as Oct4, may evoke an immune response in humans [62].
Summary
The basis of immune privilege is no longer unknown with the identification of TGF-β as one of the main choreographers; however, immune privilege is achieved by multiple factors and with combination of various mechanisms that act together in a delicately balanced way. Any disturbance to this balanced system can result in various immune disorders. Therefore, to restore immune surveillance and promote tumor eradication, application of local antagonists of TGF-β, such as antibodies, antisense oligodeoxynucleotides, short interfering RNA, etc., may be beneficial for clinical trials. Questions remain as to whether SCs are really immunogenic, since one of the main hurdles to stem cell therapies continues to be the potential for immunological recognition and rejection of the transplant by the recipient immune system. Mesenchymal SCs, hematopoietic SCs, muscle SCs and neural SCs are reported to be hypoimmunogenic, and in addition, have immune modulatory activity with potential potent mechanisms to avoid allogeneic rejection. However, the exact mechanisms remain not fully unrevealed and are still under investigation. In contrast, pluripotent SC-differentiated derivatives are reported as having reduced capacity to stimulate alloimmune responses, but these cells cannot be considered as universally immune privileged. In order to evaluate the possible immune privilege of SCs, several in vitro and in vivo methods can be applied to assess their immunotoxicity. These assays include cell surface molecule expression (e.g. MHC I and FasL expression analyzed by flow cytometry), cytokine and chemokine analysis (e.g. expression of TGF-β, IL-10 by qPCR, ELISA or flow cytometry), mixed lymphocyte reactions, NK cell and serum toxicity, and “humanized” trimera mouse models. From these assays, mixed lymphocyte reaction is the classically used test for alloimmunity, in which leukocytes from two individuals are mixed together after inactivation of the donor’s cells and the host’s lymphocyte toxicity serves as a measure for allo-rejection. NK cell toxicity assay measures the cytolytic activity of NK cells against target tumor cells by (51) Cr-release and also by determining the number of NK cells in serum using flow cytometry. NK cells function can also be evaluated by the intracellular levels of perforin, granzymes, and granulysin analyzed y flow cytometry [63]. “Humanized” trimera mouse models [55] - immunodeficient mice reconstituted with human peripheral blood mononuclear cells-represent a very promising platform to study human immune responses in vivo. However, results obtained from these models need to be carefully interpreted since immune responses may be dysfunctional in these models due to T and NK cells’ defectiveness [64,65]. Still, the field lacks a convenient model in which the immunogenicity of human SCs or human SCs-derived tissues may be tested against a competent human immune system. Future approaches to manipulate immune privilege should consider various factors at multiple levels, especially when applying SCs transplantation to regenerative medicine. Moreover, revealing the mechanisms of action of SCs natural immune privilege would also be crucial for developing strategic approaches to mitigate or inhibit the immune rejection of SC transplants.References
- Cobbold SP, Adams E, Graca L, Daley S, Yates S, et al. (2006) Immune privilege induced by regulatory T cells in transplantation tolerance. Immunol Rev 213: 239-255.
- Simpson E (2006) A historical perspective on immunological privilege. Immunol Rev 213: 12-22.
- Calder PC (2006) Branched-chain amino acids and immunity. J Nutr 136: 288S-293S.
- Munn DH, Zhou M, Attwood JT, Bondarev I, Conway SJ, et al. (1998) Prevention of allogeneic fetal rejection by tryptophan catabolism. Science 281: 1191-1193.
- Ichiryu N, Fairchild PJ (2013) Immune privilege of stem cells. Methods Mol Biol 1029: 1-16.
- Riley JK, Yokoyama WM (2008) NK cell tolerance and the maternal-fetal interface. Am J Reprod Immunol 59: 371-387.
- Keir ME, Butte MJ, Freeman GJ, Sharpe AH (2008) PD-1 and its ligands in tolerance and immunity. Annu Rev Immunol 26: 677-704.
- Fishelson Z, Donin N, Zell S, Schultz S, Kirschfink M (2003) Obstacles to cancer immunotherapy: expression of membrane complement regulatory proteins (mCRPs) in tumors. Mol Immunol 40: 109-123.
- Ng TF, Lavik E, Keino H, Taylor AW, Langer RS, et al. (2007) Creating an immune-privileged site using retinal progenitor cells and biodegradable polymers. Stem Cells 25: 1552-1559.
- Reya T (2011) Illuminating immune privilege--a role for regulatory T cells in preventing rejection. N Engl J Med 365: 956-957.
- Chen W, Wahl SM (2002) TGF-beta: receptors, signaling pathways and autoimmunity. Curr Dir Autoimmun 5: 62-91.
- Li MO, Wan YY, Sanjabi S, Robertson AK, Flavell RA (2006) Transforming growth factor-beta regulation of immune responses. Annu Rev Immunol 24:99-146.
- Wahl SM, Chen W (2005) Transforming growth factor-beta-induced regulatory T cells referee inflammatory and autoimmune diseases. Arthritis Res Ther 7: 62-68.
- Li Y, Han B, Li K, Jiao LR, Habib N, et al. (1999) TGF-beta 1 inhibits HLADR and beta 2-microglobulin expression in HeLa cells induced with r-IFN. Transplant Proc 31: 2143-2145.
- Wahl SM, Chen W (2003) TGF-beta: how tolerant can it be? Immunol Res 28: 167-179.
- D’Orazio TJ, Niederkorn JY (1998) A novel role for TGF-beta and IL-10 in the induction of immune privilege. J Immunol 160: 2089-2098.
- Xu C, Yu P, Han X, Du L, Gan J, et al. (2014) TGF-beta promotes immune responses in the presence of mesenchymal stem cells. J Immunol 192: 103-109.
- Groh ME, Maitra B, Szekely E, Koc ON (2005) Human mesenchymal stem cells require monocyte-mediated activation to suppress alloreactive T cells. Exp Hematol 33: 928-934.
- Chen CR, Kang Y, Massague J (2001) Defective repression of c-myc in breast cancer cells: A loss at the core of the transforming growth factor beta growth arrest program. Proc Natl Acad Sci U S A 98: 992-999.
- Go NF, Castle BE, Barrett R, Kastelein R, Dang W, et al. (1990) Interleukin 10, a novel B cell stimulatory factor: unresponsiveness of X chromosomelinked immunodeficiency B cells. J Exp Med 172: 1625-1631.
- de Waal Malefyt R, Abrams J, Bennett B, Figdor CG, de Vries JE (1991) Interleukin 10(IL-10) inhibits cytokine synthesis by human monocytes: an autoregulatory role of IL-10 produced by monocytes. J Exp Med 174: 1209-1220.
- Muller G, Saloga J, Germann T, Bellinghausen I, Mohamadzadeh M, et al. (1994) Identification and induction of human keratinocyte-derived IL-12. J Clin Invest 94: 1799-1805.
- Thompson-Snipes L, Dhar V, Bond MW, Mosmann TR, Moore KW, et al. (1991) Interleukin 10: a novel stimulatory factor for mast cells and their progenitors. J Exp Med 173: 507-510.
- Uccelli A, Moretta L, Pistoia V (2008) Mesenchymal stem cells in health and disease. Nat Rev Immunol 8: 726-736.
- Jones S, Horwood N, Cope A, Dazzi F (2007) The antiproliferative effect of mesenchymal stem cells is a fundamental property shared by all stromal cells. J Immunol 179: 2824-2831.
- Dazzi F, Marelli-Berg FM (2008) Mesenchymal stem cells for graft-versushost disease: close encounters with T cells. Eur J Immunol 38: 1479-1482.
- Salem HK, Thiemermann C (2010) Mesenchymal stromal cells: current understanding and clinical status. Stem Cells 28: 585-596.
- Zheng J, Song C, Zhang CC (2011) A new chapter: hematopoietic stem cells are direct players in immunity. Cell Biosci 1: 33.
- Hori J, Ng TF, Shatos M, Klassen H, Streilein JW, et al. (2007) Neural progenitor cells lack immunogenicity and resist destruction as allografts. 2003. Ocul Immunol Inflamm 15: 261-273.
- Le Blanc K (2003) Immunomodulatory effects of fetal and adult mesenchymal stem cells. Cytotherapy 5: 485-489.
- Qu-Petersen Z, Deasy B, Jankowski R, Ikezawa M, Cummins J, et al. (2002) Identification of a novel population of muscle stem cells in mice: potential for muscle regeneration. J Cell Biol 157: 851-864.
- Kolf CM, Cho E, Tuan RS (2007) Mesenchymal stromal cells. Biology of adult mesenchymal stem cells: regulation of niche, self-renewal and differentiation. Arthritis Res Ther 9: 204.
- Di Nicola M, Carlo-Stella C, Magni M, Milanesi M, Longoni PD, et al. (2002) Human bone marrow stromal cells suppress T-lymphocyte proliferation induced by cellular or nonspecific mitogenic stimuli. Blood 99: 3838-3843.
- Bartholomew A, Sturgeon C, Siatskas M, Ferrer K, McIntosh K, et al. (2002) Mesenchymal stem cells suppress lymphocyte proliferation in vitro and prolong skin graft survival in vivo. Exp Hematol 30: 42-48.
- Ramasamy R, Lam EW, Soeiro I, Tisato V, Bonnet D, et al. (2007) Mesenchymal stem cells inhibit proliferation and apoptosis of tumor cells: impact on in vivo tumor growth. Leukemia 21: 304-310.
- Sheng H, Wang Y, Jin Y, Zhang Q, Zhang Y, et al. (2008) A critical role of IFNgamma in priming MSC-mediated suppression of T cell proliferation through up-regulation of B7-H1. Cell Res 18: 846-857.
- Polchert D, Sobinsky J, Douglas G, Kidd M, Moadsiri A, et al. (2008) IFNgamma activation of mesenchymal stem cells for treatment and prevention of graft versus host disease. Eur J Immunol 38: 1745-1755.
- Hiyama A, Mochida J, Iwashina T, Omi H, Watanabe T, et al. (2008) Transplantation of mesenchymal stem cells in a canine disc degeneration model. J Orthop Res 26: 589-600.
- Ankrum JA, Ong JF, Karp JM (2014) Mesenchymal stem cells: immune evasive, not immune privileged. Nat Biotechnol.
- Bryder D, Rossi DJ, Weissman IL (2006) Hematopoietic stem cells: the paradigmatic tissue-specific stem cell. Am J Pathol 169: 338-346.
- Fujisaki J, Wu J, Carlson AL, Silberstein L, Putheti P, et al. (2011) In vivo imaging of Treg cells providing immune privilege to the haematopoietic stemcell niche. Nature 474: 216-219.
- King KY, Goodell MA (2011) Inflammatory modulation of HSCs: viewing the HSC as a foundation for the immune response. Nat Rev Immunol 11: 685-692.
- Zhang CC (2012) Hematopoietic stem cells: interplay with immunity. Am J Blood Res 2: 219-227.
- Yin H, Price F, Rudnicki MA (2013) Satellite cells and the muscle stem cell niche. Physiol Rev 93: 23-67.
- Tedesco FS, Dellavalle A, Diaz-Manera J, Messina G, Cossu G (2010) Repairing skeletal muscle: regenerative potential of skeletal muscle stem cells. J Clin Invest 120: 11-19.
- Buckingham M, Montarras D (2008) Skeletal muscle stem cells. Curr Opin Genet Dev 18: 330-336.
- Jankowski RJ, Haluszczak C, Trucco M, Huard J (2001) Flow cytometric characterization of myogenic cell populations obtained via the preplate technique: potential for rapid isolation of muscle-derived stem cells. Hum Gene Ther 12: 619-628.
- Seale P, Sabourin LA, Girgis-Gabardo A, Mansouri A, Gruss P, et al. (2000) Pax7 is required for the specification of myogenic satellite cells. Cell 102:777-786.
- Hori J, Ng TF, Shatos M, Klassen H, Streilein JW, et al. (2003) Neural progenitor cells lack immunogenicity and resist destruction as allografts. Stem Cells 21: 405-416.
- Chidgey AP, Boyd RL (2008) Immune privilege for stem cells: not as simple as it looked. Cell Stem Cell 3: 357-358.
- Li L, Baroja ML, Majumdar A, Chadwick K, Rouleau A, et al. (2004) Human embryonic stem cells possess immune-privileged properties. Stem Cells 22: 448-456.
- Koch CA, Geraldes P, Platt JL (2008) Immunosuppression by embryonic stem cells. Stem Cells 26: 89-98.
- Min JY, Yang Y, Sullivan MF, Ke Q, Converso KL, et al. (2003) Long-term improvement of cardiac function in rats after infarction by transplantation of embryonic stem cells. J Thorac Cardiovasc Surg 125: 361-369.
- Menard C, Hagege AA, Agbulut O, Barro M, Morichetti MC, et al. (2005) Transplantation of cardiac-committed mouse embryonic stem cells to infarcted sheep myocardium: a preclinical study. Lancet 366: 1005-1012.
- Drukker M, Katchman H, Katz G, Even-Tov Friedman S, Shezen E, et al. (2006) Human embryonic stem cells and their differentiated derivatives are less susceptible to immune rejection than adult cells. Stem Cells 24: 221-229.
- Grinnemo KH, Sylven C, Hovatta O, Dellgren G, Corbascio M (2008) Immunogenicity of human embryonic stem cells. Cell Tissue Res 331: 67-78.
- Swijnenburg RJ, Schrepfer S, Govaert JA, Cao F, Ransohoff K, et al. (2008) Immunosuppressive therapy mitigates immunological rejection of human embryonic stem cell xenografts. Proc Natl Acad Sci U S A 105: 12991-12996.
- Zhao T, Zhang ZN, Rong Z, Xu Y (2011) Immunogenicity of induced pluripotent stem cells. Nature 474: 212-215.
- English K, Wood KJ (2011) Immunogenicity of embryonic stem cell-derived progenitors after transplantation. Curr Opin Organ Transplant 16: 90-95.
- Okita K, Nagata N, Yamanaka S (2011) Immunogenicity of induced pluripotent stem cells. Circ Res 109: 720-721.
- Swijnenburg RJ, Schrepfer S, Cao F, Pearl JI, Xie X, et al. (2008) In vivo imaging of embryonic stem cells reveals patterns of survival and immune rejection following transplantation. Stem Cells Dev 17: 1023-1029.
- Dhodapkar KM, Feldman D, Matthews P, Radfar S, Pickering R, et al. (2010) Natural immunity to pluripotency antigen OCT4 in humans. Proc Natl Acad Sci U S A 107: 8718-8723.
- Li Q (2010) NK cell assays in immunotoxicity testing. Methods Mol Biol 598: 207-219.
- Watanabe Y, Takahashi T, Okajima A, Shiokawa M, Ishii N, et al. (2009) The analysis of the functions of human B and T cells in humanized NOD/shi-scid/gammac(null) (NOG) mice (hu-HSC NOG mice). Int Immunol 21: 843-858.
- Andre MC, Erbacher A, Gille C, Schmauke V, Goecke B, et al. (2010) Long-term human CD34+ stem cell-engrafted nonobese diabetic/SCID/IL-2R gamma(null) mice show impaired CD8+ T cell maintenance and a functional arrest of immature NK cells. J Immunol 185: 2710-2720.