Journal of Bioelectronics and Nanotechnology
Download PDF
Review Article
*Address for Correspondence: Mohit Rawat, Department of Nanotechnology, Sri Guru Granth Sahib World University, Fatehgarh Sahib 140406, Punjab, India, Tel: +91 8872108007; E-mail: mohitnano.nit@gmail.com
Citation: Kaur N, Kaur S, Singh J, Rawat M. A Review on Zinc Sulphide Nanoparticles: From Synthesis, Properties to Applications. J Bioelectron Nanotechnol 2016;1(1): 5.
Copyright © 2016 Kaur N, et al. This is an open access article distributed under the Creative Commons Attribution License, which permits unrestricted use, distribution, and reproduction in any medium, provided the original work is properly cited.
Journal of Bioelectronics and Nanotechnology | Volume: 1, Issue: 1
Submission: 10 October, 2016 | Accepted: 15 December, 2016 | Published: 20 December, 2016
Kanemeto et al. have reported the photo-physical and photocatalytic properties of ZnS nanoparticles and explained the role of defect levels in influencing the luminescence characteristics of quantum particles. PL studies revealed a peak at 325 nm (3.82 eV) which was due to transition of trapped electrons to valence levels. Thus it was concluded that capping agents are important in removing Zn dangling orbitals (electron traps) from the gap. Observation of broad red shift in luminescence and absence of band edge luminescence is due to unsaturated sp3 hybridized orbitals of surface S atoms. In this paper they have measured PL emission for various excitation wavelengths. They observed a broad peak at about 363 nm (3.41 eV) for all samples in the PL spectra for all organic passivating agents at 220 nm excitation wavelength [2].
J.F. Xu et al prepared Zn nanoparticles the inert-gas evaporation technique with induction heating method. As prepared Zn particles were mixed with Na2S solution. ZnS nanoparticles were synthesized under ultrasonic radiation. TEM observations showed that the ZnS nanoparticles are in a spherical form with an average diameter of 40 nm (Figure 5) [10].
A Review on Zinc Sulphide Nanoparticles: From Synthesis, Properties to Applications
Navneet Kaur, Sukhmeen Kaur, Jagpreet Singh and Mohit Rawat*
- Department of Nanotechnology, Sri Guru Granth Sahib World University, Fatehgarh Sahib, Punjab, India
*Address for Correspondence: Mohit Rawat, Department of Nanotechnology, Sri Guru Granth Sahib World University, Fatehgarh Sahib 140406, Punjab, India, Tel: +91 8872108007; E-mail: mohitnano.nit@gmail.com
Citation: Kaur N, Kaur S, Singh J, Rawat M. A Review on Zinc Sulphide Nanoparticles: From Synthesis, Properties to Applications. J Bioelectron Nanotechnol 2016;1(1): 5.
Copyright © 2016 Kaur N, et al. This is an open access article distributed under the Creative Commons Attribution License, which permits unrestricted use, distribution, and reproduction in any medium, provided the original work is properly cited.
Journal of Bioelectronics and Nanotechnology | Volume: 1, Issue: 1
Submission: 10 October, 2016 | Accepted: 15 December, 2016 | Published: 20 December, 2016
Abstract
In this paper the synthesis of zinc sulfide nanoparticles by different routes has been discussed. The emergence of nanotechnology and the synthesis of nanomaterials have revolutionized the field of science and technology. ZnS tends to show the most promising nanoscale morphologies among the inorganic semiconductors thus possessing versatile novel properties and applications. These applications include their use in Field Emitting Diodes (FET), electroluminescence, sensors (UV-light sensors, gas sensors, biosensors), flat panel displays. This article begins with the brief introduction to ZnS its structure, description of physical, chemical and electronic properties. Further followed by the recent experiments conducted in order to improve its nanoscale properties and thus idealizing its applications.Keywords
Zinc sulphide; Electroluminescence; SensorsIntroduction
NanotechnologyThe emergence of nanotechnology is often credited to renowned Physicist Richard Feynman’s talk, entitled “There’s Plenty of Room at the Bottom: Invitation to Enter a New Field of Physics” held at an American Physical Society meeting at the California Institute of Technology in 1959. A nanometer is a one billionth of a meter i.e. 10-9 m. The nanomaterials exhibit distinctive physical properties as compared to the bulk. The size dependent properties at this scale make nanomaterials capable of enhancing the performance and shelf life of number of products in the industrial sector.
Zinc sulphide
ZnS is one of the first semiconductors discovered that has shown the remarkable properties that can be exploited for versatile applications including field emitters, electroluminescence, electro catalyst, biosensors. Compared to bulk ZnS, nano ZnS possess anomalous physical and chemical properties such as: enhanced surface to volume ratio, the quantum size effect, surface and volume effect and macroscopic quantum tunneling effect, more optical absorption, chemical activity and thermal resistance, catalysis, and the low melting point.
Crystal structure
Zinc Sulphide (ZnS) is a very important II-VI semiconductor that has been researched widely due to its broad spectrum of potential applications. ZnS is chemically much stable as well as technologically much better than its alternative chalcogenides (such as ZnSe), hence is considered as a favourable host material. ZnS is a polymorphous material that is it exist in two crystalline forms namely Zinc Blend (sphalerite) and Wurtzite. In both forms the co-ordination geometry at Zn and S is tetrahedral. Zinc blend has more stable cubic form (fcc) whereas wurtzite has hexagonal form (hcp). ZnS exhibit large band gap of approximately 3.54 eV and 3.91 eV for zinc blend and wurtzite respectively. Due to its large band gap than ZnO (3.4 eV) it is considered more suitable for Visible and UV light based devices. Both structures have similar closest neighbour connections but the distances and angles to furthermore neighboru’s quietly differ. Zinc blend has four asymmetric units in its unit cell whereas wurtzite has 2.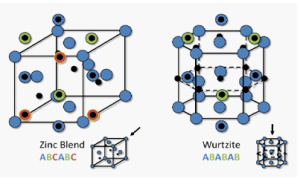
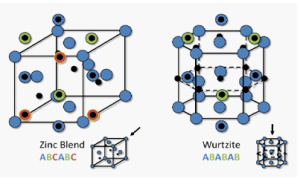
Synthesis Overview
Ram Kripal et al., prepared ZnS: Mn+2 by co-precipitation method and conducted their photo luminescent and photoconductivity properties. UV- visible spectra shows blue shift as compared to bulk counterpart. PL spectra show orange emission that varies with Mn+2 concentrations. The XRD studies estimated the size of nanoparticles to be around 2-4 nm. The TEM images overestimates the larger nanoparicle size due to drying step in sample preparation. The time resolved rise and decay of photocurrent indicates the anomalous behaviour during steady state illumination (Figure 1) [1]
Kanemeto et al. have reported the photo-physical and photocatalytic properties of ZnS nanoparticles and explained the role of defect levels in influencing the luminescence characteristics of quantum particles. PL studies revealed a peak at 325 nm (3.82 eV) which was due to transition of trapped electrons to valence levels. Thus it was concluded that capping agents are important in removing Zn dangling orbitals (electron traps) from the gap. Observation of broad red shift in luminescence and absence of band edge luminescence is due to unsaturated sp3 hybridized orbitals of surface S atoms. In this paper they have measured PL emission for various excitation wavelengths. They observed a broad peak at about 363 nm (3.41 eV) for all samples in the PL spectra for all organic passivating agents at 220 nm excitation wavelength [2].
Manzoor et al. studied multi color emitting doped ZnS nanocrystals by making use of pyridine (P-ZnS) or Polyvinyl Pyrrolidone (PVPZnS) as capping agents via wet chemical methods. The PL studies showed that the emission related to dopant from P-ZnS nanocrystals are due to energy transfer during band-to-band excitation of the host lattice. Although, in the case of PVP-ZnS, significant enrichment in the emission intensity was observed and the corresponding excitation spectra appeared to be broadened due to multiple excitation bands having peak maxima at 235, 253, 260, 275, and 310 nm. The presence of PVP related energy bands in the excitation spectra describe the process of energy transfer from the surface adsorbed PVP molecules to dopant centers in ZnS nanocrystals. Hence the study brings out a heterogeneous sensitizer-activator relationship between organic adsorbate and doped semi conductor nanocrystals [3].
Manoj et al. reported the study of energy transfer mechanism using different capping agents to intrinsic luminescent vacancy centers of ZnS. Co-precipitation method was used to synthesize nanoparticles of capped and uncapped ZnS. Sterically stabilized NPs were obtained using organic polymers: poly vinyl pyrrolidone, thioglycerol and 2-mercaptoethanol. TEM observation revealed that Monodispersed NPs were observed for both capped and uncapped ZnS nanopowders. Though, tendency of forming nanorod like structures existed for nanopowders of uncapped ZnS. X-ray diffraction pattern gave the size in between 1.95-2.20 nm for capped nanostructures and 2.2 nm for uncapped nanostructures. Emission intensity and Band gap were observed to be increased on addition of different capping agents in comparison to uncapped ZnS NPs. Overall result revealed that Capped ZnS NPs showed more pronounced energy transfer from capping layer to photoluminescent [4].
Gilbert et al. combined real space Pair Distribution Function (PDF) analysis of nanoparticle structure with the particle size analysis (zetasizer) and FTIR to point out that the nature of surface solvent or ligand interactions will have an effect on the interior crystallinity of nanoparticles. The availability of particles with a variety of crystallinity provides a model system during which it’s possible to see the interior disorder effects of nanoparticle, where properties like mechanical stiffness and fluorescent quantum yield are affected. PDF analysis showed that samples of ZnS nanoparticle with similar mean diameters (3.2-3.6 nm) however synthesized and treated differently possess a dramatic range of interior disorder [5].
Ghosh et al. synthesized Polyvinyl Pyrrolidone (PVP) encapsulated Zinc Sulphide (ZnS) nanoparticles by adding measured amount of zinc acetate, thiourea and PVP into N, N- Dimethyl Formamide (DMF) medium with consistent stirring at 150 rpm. The resulted TEM image of ZnS NPs explained the effect of capping on size separation. It exhibited that PVP capped ZnS nanoparticles were mono dispersed in nature. Their size ranging within 2-3 nm matches with of XRD results. Whereas uncapped nanoparticles on the other hand were aggregated and were larger in sizes than capped ones. This explains the use of PVP as capping agent. Also Blue shift in absorption edge as compared to bulk ZnS clearly explained the quantum confinement effect within ZnS nanoparticles [6].
Vacassy et al. fabricated ZnS nanoparticles in the powder form by using precipitation method from homogeneous solutions of various zinc compounds, with S2- as precipitating anion, formed by decomposition of thioacetamide. They observed that nucleation is enhanced by the use of acetic acid but restricted particle growth occurs due to formation of complex with zinc cations that lowers the concentration of free cations in the solution. Also, the complexing attachment phenomena of the ZnS particles with acetate and acetylacetonate anions leading to the seize of particle growth processes. The presence of complexed Zn2+ species in the acidic solution is demonstrated both theoretically (using a model based on the calculations of the solubility isotherms of the soluble species) and experimentally by Fourier-transform infrared techniques [7].
Somayeh Nazerdeylami et al. synthesized ZnS: Mn+2 nanoparticles capped with PVP in aqueous solution by chemical method. Prepared samples were characterized by using UV-Visible spectroscopy, XRD and PL studies. The UV data reveals that the synthesized nanoparticles show absorption near 292 nm and also that the concentration of Mn oes not alter the bang gap of nanoparticles. XRD studies show that the average particle size is 2 nm and ZnS nanoparticles cubic structure. PL studies performed at room temperature show the orange-red emission at 594 nm and its intensity increases with increase in Mn+2 ion concentration (Figures 2 & 3) [8].
Sarika Pandey et al. prepared Manganese doped ZnS nanoparticles capped with histidine molecule by co-precipitation reaction from the homogenous solution of zinc and manganese salts. The PL spectrum shows the emission peak of doped nanoparticles at around 590 nm. The XRD results calculated the average particle size of ZnS nanophosphor by Debye Scherrer’s formula to be of the order of 5-6 nm. A small angle X-ray study shows the maximum uniform particle size distribution of 3.5 nm for the prepared sample (Figure 4) [9].
J.F. Xu et al prepared Zn nanoparticles the inert-gas evaporation technique with induction heating method. As prepared Zn particles were mixed with Na2S solution. ZnS nanoparticles were synthesized under ultrasonic radiation. TEM observations showed that the ZnS nanoparticles are in a spherical form with an average diameter of 40 nm (Figure 5) [10].
Doping of Semiconductors
To modify the properties of intrinsic semiconductors they need to be doped. The doping concentration generally around one dopant atom per 105 atoms. The semiconductors are doped with impurities to create impurity centres that interact with electrons and holes and due to this interaction the middle gap states arising from surface species are shifted outside the gap region. The absorption a spectrum is not affected by doping however, the emission intensity is significantly enhanced. The doping is simply achieved by addition of the dopant into the reaction mixture. If the impurity-induced transition can be restricted as in the case of the transition metals or the rare earth elements, the radiative efficiency of the impurity-induced emission increases significantly. Thus doped nanomaterials make a significant contribution in the field of electronics, photonics, optics and medical sciences.Doped zinc sulphide
ZnS nanoparticles have high surface to volume ratio thus exhibit large band gap and hence are more promising carriers of photoluminescence [11]. In order to use the semiconductor nanocrystals for the fabrication and commercialization of devices at nanoscale, it is required to synthesize nanoparticals with different physical and chemical properties and this can be achieved by doping. The dopants form the impurity centres that interact with the electrons and hole. The addition of impurities shift the central recombination from surface states to that of impurity states thus enhancing the radiative efficiency. ZnS nanoparticles doped with transition metal ions are the most important material for research in semiconductor nanocrystals and suggest a new class of luminescent materials. The Mn+2 ion have the electronic configuration [Ar] 3d5, these d states of Mn+2 hybridize with the s-p states of ZnS lattice thus resulting in faster energy transition between the two,hence increasing the quantum efficiency. The Mn+2 ions have the broad emission peak that depends upon the host lattice [12-15].
Potential Applications
Field Emission (FE)FE is the phenomenon defined as the emission of electron induced by external electromagnetic field. As ZnS nanostructures with excellent crystallinity can be obtained in high field, their uses in FET based devices have been demonstrated. ZnS is believed to be a promising field emission material since it has a very large work function (~7.0 eV) than the other popular materials like Si (~3.6 eV), WO3(5.7 eV), ZnO (5.3 eV). ZnS nanobelts/nanoribbons/nanosheets exhibit enhanced field emission performance including emission current, field-enhancement factors, stability, turn on field. This is achieved by increasing their aspect ratio (length to thickness ratio), assembling them into arrays or decorating their surface with a low work function material. The smallest width of nanobelts found is ~7.5 nm. These extremely thin nanobelts possess pronounced quantum confinement effect. Also no current degradation or notable fluctuations are observed with these structural arrays [16].
Catalytic activities
ZnS is a direct band gap material with astonishing chemical stability against oxidation and hydrolysis. All these characteristics are maintained when the material is featured to nano dimensions therefore nanoparticles of ZnS are interesting entities for catalytic applications where they are exposed to violent environment. Moreover ZnS is available in abundance in nature and is non toxic. Therefore they can be used as an important catalyst in environmental protection by removing organic and toxic water pollutants. ZnS have been used for the photocatalytic degradation of organic pollutants such as dyes, p-nitrophenol and halogenated benzene derivatives in waste water treatment. The photocatalytic superiority of ZnS nanoporous nanoparticles is due to the fact that due to their excellent porous structures they do not aggregate. Moreover ZnS can be doped with Ni or Cu to adjust the photocatalytic activity under visible irradiation. ZnS nanoparticles have also been used as an electrocatalyst for the direct conversion of ethanol in fuel cells [17].
Sensors
UV-Sensors: ZnS makes a promising material for fabricating optoelectronic devices. ZnS provides a novel forthcoming alternative for UV detectors due to its wide direct band gap (~3.7 eV) as compared to that of ZnO and indirect band gap of diamond (~5.5 eV). The photoresponse of ZnS nanobelts based sensors is three orders of magnitude higher upon illumination at 320nm light as compared to its response to visible light. The high spectral selectivity along with high photosensitivity and fast response time justify the use of ZnS nanobelts as a promising UV photodetectors [18].
Chemical Sensors: ZnS nanocrystals have a very good photostability, continuous absorption spectra, efficient, narrow and tunable emission, which can be exploited or their application in biological imaging and single particle tracking studies. Mn+2 doped ZnS nanocrystals with amine capping layer have been fabricated and utilized for the fluorescence detection of chemicals like 2,4,6-trnitrotoluene (TNT) by quenching the strong orange Mn+2 photoluminescence [19].
Biosensors: ZnS nanoparticles have been used for label free, real time and sensitive detection of biological species. ZnS QDs are luminescent inorganic fluorophores that have the potential to overcome the functional limitations possessed by organic dyes in fluorescence labelling applications. To be a promising candidate for biosensors, the nanoparticles must have high luminescent efficiency and appropriate surface groups available in order to couple with the biomolecules. Recently CdSe/ZnS core-shell QDs conjugated with enzymes is used to sense glucose. The QDs were used as electron donors whereas enzymes were used as acceptors for oxidation/ reductions reactions involved in oxidizing glucose to gluconic acid. The newly developed QD systems possess superior design, high flexibility, low cost and good sensitivity [20,21].
Glucose biosensor: A novel electrochemical glucose biosensor was developed by depositing an Ionic Liquid (IL) (e.g., 1-ethyl-3-methylimidazolium trifluoromethanesulfonate; [EMIM] [Otf]), ZnO nanoparticles (ZnO NPs) and Eggshell Membrane (ESM) on a modified Glassy Carbon Electrode (GCE) for determination of glucose [22]. Enzymatic glucose biosensor was fabricated with the aid of zinc oxide (ZnO) porous nanostructure on FTO glass substrate by simple drop casting technique. By introducing a small layer of Platinum (Pt) nanopartides and Chitosan (CS) over the ZnO porous nanostructure have enhanced the glucose sensing property of the fabricated biosensor to a higher order. The morphological and structural properties of the electrodes were analyzed using scanning electron microscopy and X-ray diffraction analysis. The glucose sensing properties of Glucose Oxidase (GOx) immobilized electrodes were estimated using electrochemical analysis. The ZnO/Pt/CS hetero structure conjugated with GOx shows good analytical performances with high sensitivity in a wide linear range and low detection compared with the bare ZnO/CS/GOx biosensor (Figure 6) [23].
Conclusion
In this review article the fabrication of ZnS nanoparticles doped with Mn and other transition metals by various methods such as chemical precipitation, sol-gel, hydrothermal, inert-gas evaporation etc have been studied. The synthesized nanoparticles were being characterized by using XRD, TEM, HRSEM, UV-Visible spectroscopy, FTIR, Zeta-sizer and PL spectroscopy. Doping of ZnS with Mn alters the band structure, optical and chemical properties and enhances the luminescence properties. These materials are non toxic so they can be further elaborated for their application in biosensing and imaging.References
- Kripal R, Gupta AK, Mishra SK, Srivastava RK, Pandey AC, et al. (2010) Photoluminescence and photoconductivity of ZnS: Mn2+ nanoparticles synthesized via co-precipitation method. Spectrochim Acta A Mol Biomol Spectrosc 76: 523-530.
- Khosravi AA, Kundu M, Kuruvilla BA, Shekhawat GS, Gupta RP, et al. (1995) Manganese-Doped Zinc-Sulfide nanoparticles by aqueous method. Appl Phys Lett 67: 2506-2508.
- Manzoor K, Vadera SR, Kumar N, Kutty TR (2004) Multicolor electroluminescent devices using doped ZnS nanocrystals. Appl Phys Lett 84: 284-286.
- Sharma M, Kumar S, Pandey OP (2010) Study of energy transfer from capping agents to intrinsic vacancies/defects in passivated ZnS nanoparticles. J Nanopart Res 12: 2655-2666.
- Gilbert B, Huang F, Lin Z, Goodell C, Zhang H, et al. (2006) Surface chemistry controls crystallinity of ZnS nanoparticles. Nano Lett 6: 605-610.
- Ghosh G, Naskar MK, Patra A, Chatterjee M (2006) Synthesis and characterization of PVP-encapsulated ZnS nanoparticles. Opt Mater 28: 1047-1053.
- Vacassy R, Scholz SM, Dutta J, Plummer CJ, Houriet R, et al. (1998) Synthesis of controlled spherical zinc sulfide particles by precipitation from homogeneous solutions. J Am Ceram Soc 81: 2699-2705.
- Nazerdeylami S, Saievar-Iranizad E, Dehghani Z, Molaei M (2011) Synthesis and photoluminescent and nonlinear optical properties of manganese doped ZnS nanoparticles. Physica B Condens Matter 406: 108-111.
- Pandey S, Verma P, Pandey AC (2006) Synthesis of highly luminescent manganese doped ZnS Nanophosphor. Proc ASID 259-261.
- Xu JF, Ji W, Lin JY, Tang SH, Du YW (1998) Preparation of ZnS nanoparticles by ultrasonic radiation method. Appl Phys A 66: 639-641.
- Bhargava RN, Gallagher D, Hong X, Nurmikko A (1994) Optical properties of manganese-doped of ZnS. Phys Rev Lett 72: 3-17.
- Bol AA, Meijerink A (1998) Long-lived Mn2+ emission in nanocrystalline ZnS/Mn2+. Phys Rev B 58: R15997-R16000.
- Smith BA, Zhang JZ, Joly A, Liu J (2000) Luminescence decay kinetics of Mn2+ doped ZnS nanoclusters grown in reverse micelles. Phys Rev B 62: 2021-2028.
- van Sark WG, Frederix PL, Van den Heuvel, Gerritsen DJ, Bol HC, et al. (2001) Photooxidation and photobleaching of single cdse/zns quantum dots probed by room-temperature time-resolved spectroscopy. J Phys Chem B 105: 8281-8284.
- Murase N, Jagannathan R, Kanematsu Y, Watanabe M, Kurita A, et al. (1999) Fluorescence and epr characteristics of Mn2+ -doped zns nanocrystals prepared by aqueous colloidal method. J Phys Chem B 103: 754-760.
- Zhi-Gang C, Zou J, Dai-Wei W, Li-Chang Y, Liu G, et al. (2009) Field emission and cathodoluminescence of zns hexagonal pyramids of zinc blende structured single crystals. Adv Funct Mater 19: 484-490.
- Naeimi H, Kiani F, Moradian M (2014) ZnS nanoparticles as an efficient and reusable heterogeneous catalyst for synthesis of 1-substituted-1H-tetrazoles under solvent-free conditions. J Nanopart Res 16: 1-9.
- Fang X, Band Y, Gautam UK, Zhai T, Zeng H, et al. (2009) ZnO and ZnS nanostructures: ultraviolet-light emitters, lasers, and sensors. Crit Rev Solid State Mate Sci 34: 190-223.
- Wang X, Xie Z, Huang H, Liu Z, Chen D, et al. (2012) Gas sensors, thermistor and photodetector based on ZnS nanowires. J Mater Chem 22: 6845-6850.
- Zhang F, Li C, Li X, Wang X, Wan Q, et al. (2006) ZnS quantum dots derived a reagentless uric acid biosensor. Talanta 68: 1353-1358.
- Wurtzite crystal structure. Wikipedia.
- Aini BN, Siddiquee S, Ampon K, Rodrigues KF, Suryani S (2015) Development of glucose biosensor based on ZnO nanoparticles film and glucose oxidase-immobilized eggshell membrane, Sens Biosensing Res 4: 46-56.
- Anusha JR, Hee-Je Kim, Fleming AT, Jerome Das S, Kook-Hyun Y, et al. (2014) Simple fabrication of ZnO/Pt/chitosan electrode for enzymatic glucose biosensor. Sens Actuators B Chem 202: 827-833.